How would quantum information science shape communications technology?
A quantum is the smallest unit of things. Things at the quantum scale, such as clusters of atoms and subatomic particles, behave differently than they do in our everyday macroscopic world. Scientists are taking advantage of these differences to develop devices that can store, share, and compute information faster. At the Institute of Applied Physics and Materials Engineering (IAPME) of the University of Macau (UM), Chair Professor Tang Zikang, who is the director of IAPME, Associate Professor Ian Hou, Assistant Professor Lai Shen and Assistant Professor Sun Pengzhan have formed the Quantum Information Research Group. Together they are exploring new ways of processing information that use the principles of quantum mechanics, in the hope of advancing fields such as computing and communications technology.
Exploring the quantum realm
China’s 14th Five-Year Plan, which was released in 2021, sets out plans for a variety of nationwide science and technology initiatives with a focus on emerging fields such as quantum information science (QIS). In the United States, federal funding for quantum information research has grown significantly since the introduction of the National Quantum Initiative Act in 2018. Countries including Germany, Japan, and the United Kingdom have also developed strategies to promote quantum technology.
Researchers around the world are harnessing this trend with advanced technologies to develop quantum computing, one of the most important applications of QIS. Quantum computers can perform multiple computations simultaneously, unlike classical computers which can only perform one computation at a time. These novel computers store data in qubits, or quantum bits, which can represent not only 0 or 1, but also a unique ‘superposition’ state with different ratios, in which 0 and 1 exist at the same time.
In front of a whiteboard brimming with mathematical equations in his office, Prof Tang, a physicist who developed the world’s smallest single-walled carbon nanotubes, explains why researchers at UM are exploring quantum information science (QIS) processing with classical coherent light. ‘Quantum computing has limitless potential to perform computations to solve challenges that classical computers take years to solve or even cannot solve,’ he says. ‘As a result, quantum computing has become a subject of great interest worldwide. At UM, our team is striving to make breakthroughs in areas of global interest.’ To achieve this goal, the Quantum Information Research Group has been working on a brand-new materials system based on quantum information and computation, nonlinear quantum transport, and topological topics in solid-state theory, striving for cutting-edge discoveries.
To achieve this goal, Prof Tang and three other physicists from IAPME, namely Associate Professor Ian Hou, as well as Assistant Professors Lai Shen and Sun Pengzhan, have formed the Quantum Information Research Group. The group works on a brand-new materials system based on quantum information and computation, nonlinear quantum transport, and topological topics in solid-state theory, striving for cutting-edge discoveries.
Quantum computing with classical coherent light
Prof Ian, a scientist with extensive experience researching quantum information processing and quantum computation at UM, explains that the development of sophisticated, fully functional quantum computers still faces enormous challenges. ‘For example, a qubit state is extremely vulnerable and sensitive and can flip its state with the slightest change in temperature. It is often extremely costly to keep the temperature of qubits close to absolute zero when manipulating and studying them,’ he says.
Prof Ian adds that in the quantum world, one particle can be connected to another particle in a strange way, and whenever the state of one of the particles changes, the state of the other particle is automatically affected, regardless of the physical distance between them. ‘This phenomenon is known as “quantum entanglement”. Quantum algorithms usually require two particles to be stably entangled, which involves a complicated technical process,’ he says.
Faced with this reality, Prof Tang and Prof Ian have been exploring the use of classical coherent optical techniques to replace the use of qubits with macroscopic states of light, which are the properties of light that can be observed at a macroscopic level. Their research led to a breakthrough when they applied this method to a highly efficient quantum algorithm for integer factorisation, the process of decomposing a large integer into two prime numbers. ‘Many scientists believed that this algorithm could only be implemented through quantum entanglement, but we have proven that what people believe is not definitely true,’ says Prof Ian.
In essence, Prof Tang and Prof Ian have used two attributes of macroscopic light – polarisation, which indicates the direction of the light, and amplitude, which measures the strength of the vibration — to achieve the function of qubits. The researchers then ‘entangled’ these two attributes of a photon so that they mirror each other, as if they are two particles in quantum entanglement.
With Shor’s algorithm, a quantum algorithm, they seek to factorise integers using photons, without the need for low-temperature environments. ‘This is much cheaper and easier than entangling two particles to use this quantum algorithm specific by quantum mechanics,’ explains Prof Ian. ‘As many cryptographic methods require factorisation of large integers, the widespread use of quantum algorithms for this purpose could profoundly improve the security and convenience of information transmission, for example in banking transactions and private messages,’ Prof Ian says.
New materials for sensors in communications devices
QIS also involves studying potential materials for next-generation sensors. Communications devices such as mobile phones, cellular stations and satellites all contain sensors. Prof Lai, an expert on a physical phenomenon known as the ‘Hall effect’, explains, ‘Certain materials exhibit a distinct Hall effect when an alternating current electric field is applied in a specific crystal direction. By measuring this voltage, communications devices can detect the surrounding electromagnetic field. For example, a sensor in a mobile phone can identify nearby wireless communication electromagnetic signals by measuring the Hall voltage.’
In recent years, terahertz communication has become increasingly important in the development of 6G mobile communications networks. Terahertz detectors are essential components of these systems and play a key role in determining their potential applications. However, current terahertz detectors have some tough issues that need to be addressed. That is why there has been growing interest in materials that exhibit the ‘nonlinear Hall effect’, which are not affected by material scattering and other external factors. These materials are expected to improve the performance and reliability of future communications devices.
A research team of which Prof Lai was once a member found that a particular type of non-linear Hall effect is caused by a material property known as the ‘Berry connection polarisability tensor’. Since joining UM, he has continued to investigate this topic. ‘I am deepening my research on this type of non-linear Hall effect, in the hope that such findings will facilitate the development of sensors for quantum communications devices that can accurately detect and absorb nearby electromagnetic signals,’ he says.
Tiny tunnels for complex information
While most electronic devices transmit information via the flow of electrons, Prof Sun has pioneered the use of molecular ion flow to do so. ‘To transmit information, I have constructed some tiny tunnels using two-dimensional materials like graphene. These tunnels can selectively allow a single molecular ion, which serves as an information carrier, to pass through,’ explains Prof Sun.
Many experts believe that the laws of classical mechanics generally do not apply to objects at the quantum scale. However, an overseas research team with which Prof Sun once collaborated made an interesting discovery. The team found that the Kelvin equation, which describes the aggregation of fluids in tiny tubes, a phenomenon known as the ‘capillary action’, also holds in the sub-nanometre-scale tunnels they constructed. ‘At UM, I continue to investigate the physical properties inside these tiny tunnels. This study could result in techniques for precisely controlling the flow of molecular ions,’ says Prof Sun.
In addition, Prof Sun has been building ‘heterogeneous’ 2D tunnels in the nanometre range and even the smaller sub-nanometre range. ‘We can build tiny tunnels with various physical properties by combining different 2D crystals the same way we put Lego bricks together. The development of these tunnels will help us to develop new conceptual means of communications that can exchange very complicated information with molecular ions,’ he adds.
Quantum research for an intelligent society
QIS promises to revolutionise key technologies in areas such as communications and computing, which are important building blocks for a more intelligent society. Looking into the future, Prof Tang says, ‘Our institute is determined to increase collaboration with other universities and institutions for QIS research. We are not only committed to developing new technologies and exploring novel physical phenomena, but also expanding the practical applications of QIS.’
Source: My UM Issue 122
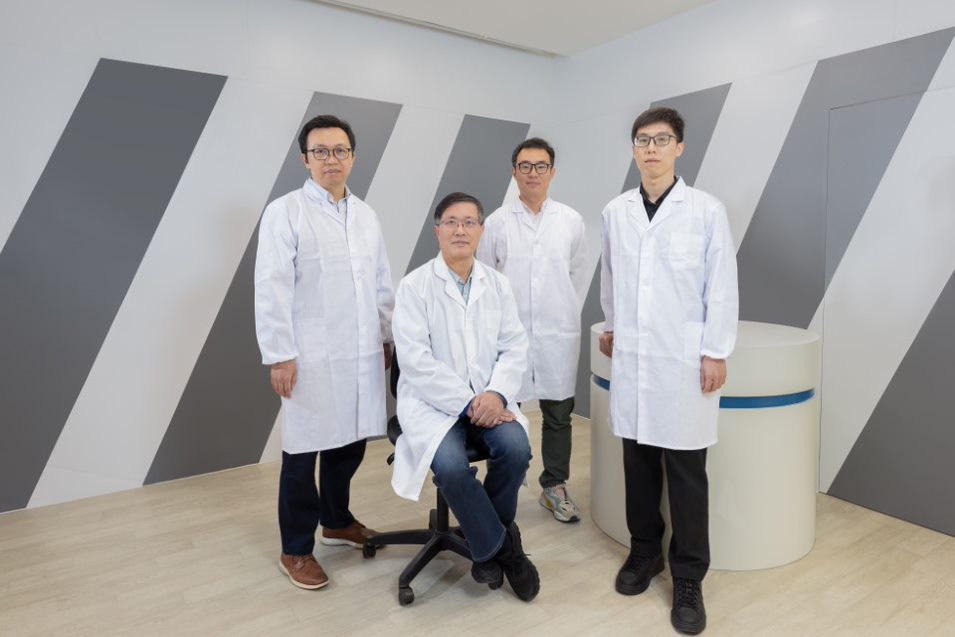
Quantum Information Research Group of the Institute of Applied Physics and Materials Engineering at UM — from Left: Prof. Hou IAN, Prof. Zikang TANG, Prof. Pengzhan SUN, and Prof. Shen LAI
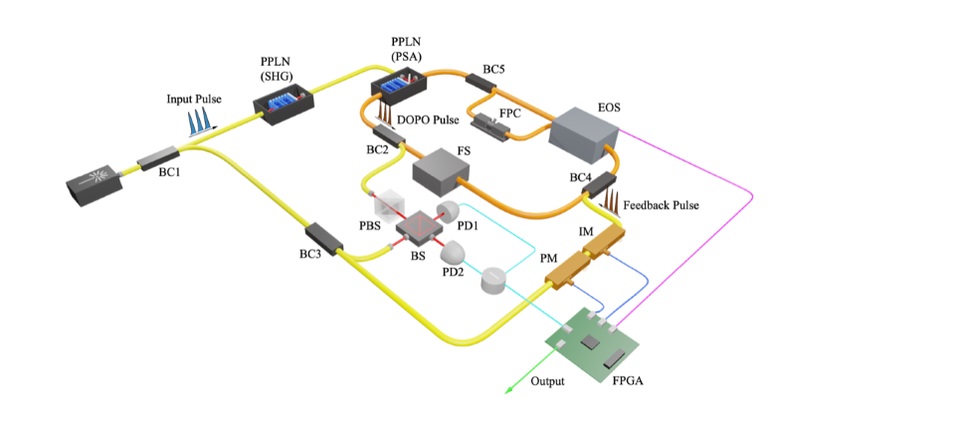
Prof. Hou IAN’s study involves using classical coherent light for quantum computing processing.
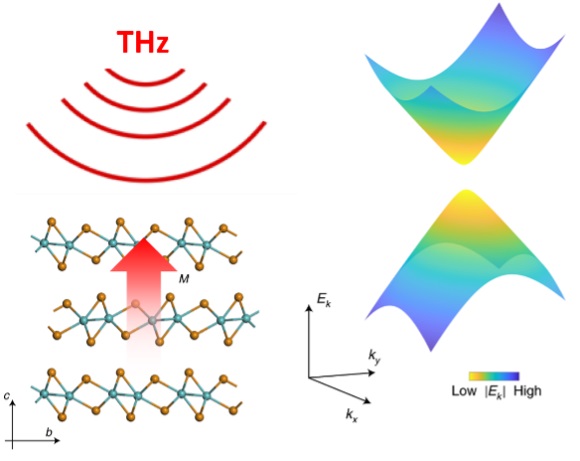
Materials that exhibit a nonlinear Hall effect caused by the “Berry connection polarisability tensor” can effectively detect electromagnetic waves in the terahertz frequency range.
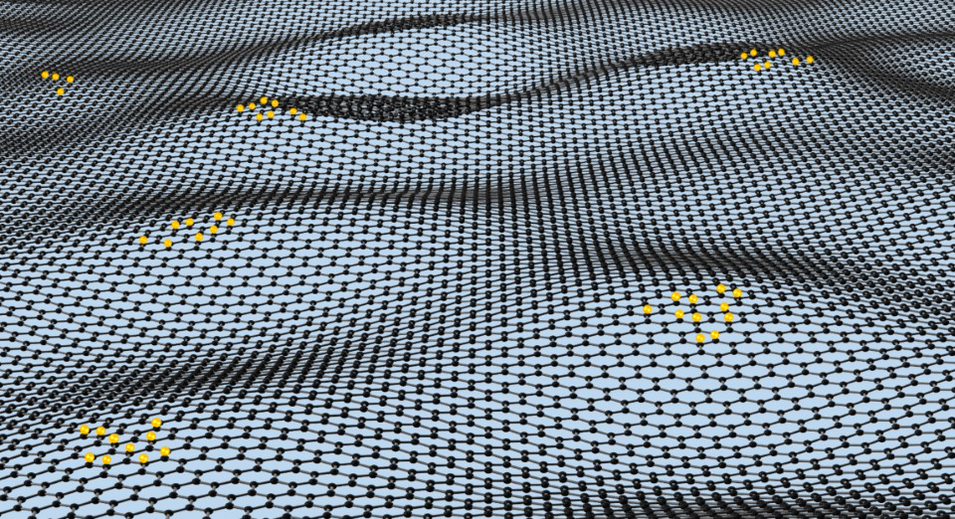
This image shows how hydrogen atoms and subatomic particles such as protons can be selectively transported through the lattice in two-dimensional materials like graphene